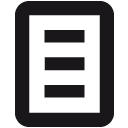
DC Element | Wert | Sprache |
---|---|---|
dc.contributor.advisor | Betzel, Christian (Prof. Dr.) | |
dc.contributor.author | Botha, Sabine | |
dc.date.accessioned | 2020-10-19T13:20:27Z | - |
dc.date.available | 2020-10-19T13:20:27Z | - |
dc.date.issued | 2018 | |
dc.identifier.uri | https://ediss.sub.uni-hamburg.de/handle/ediss/7675 | - |
dc.description.abstract | Structural biologist strive towards the ability of imaging single biological particles such as viruses, ribosomes or even single protein molecules with atomic resolution without the need for tedious sample preparation or crystallization. Organic matter is however highly sensitive to radiation damage and therefore severely limits the imaging capabilities of available techniques, such as electron microscopy or X-ray diffraction analysis. Since the advent of free electron lasers (FELs), this has become theoretically realizable, and therefore methods towards using coherent diffractive imaging approaches using an FEL have been undergoing development during the past decade. The theory is based upon the ”diffraction before destruction” principle, which states that when using an ultra-short, yet highly brilliant X-ray pulse, as generated at an FEL, the diffraction pattern from a single biological particle can be collected before the radiation damage induced by the X-rays traverses and obliterates the sample. These experiments, however, require the experiment to take place under vacuum conditions, and therefore the particles to be analyzed need to be highly uniform and homogeneous in solution and subsequently dried free of remnants, since thousands of individual diffraction patterns in random orientations are merged into a 3D diffraction volume. To this end, two virus samples which were deemed suitable for method and algorithm development, were characterized and prepared for single particle coherent diffractive imaging experiments at an FEL during the course of this work. The analyzed viruses were a dsDNA-adenovirus, and a ds-RNA Mycovirus. Furthermore small angle X-ray scattering experiments were performed at a synchrotron, and first structural insights into the viruses could be obtained. A further application of the ”diffraction before destruction” approach is serial crystallography. The most commonly used method for solving protein structures is macromolecular crystallography. The long established rotational method poses the barrier of having to grow large protein crystals. For serial crystallography approaches, crystals that are too small for conventional, rotational X-ray diffraction structure solution can be applied. Instead of collecting one complete data set from a single crystal, only a single diffraction pattern is collected per crystal which are then merged into a complete dataset. The successful application of this method for structure solution has been demonstrated numerous times at FELs as well as initial proof of principle experiments at synchrotron X-ray sources. In addition to the benefits of being able to apply small crystals, data collection is also done at room temperature, which is usually not possible applying single crystal diffraction due to the implications this has for the increase in radiation damage. This makes it possible for scientists to probe proteins close to physiological conditions, and sets the path for time-resolved studies of the proteins. Such experiments are however not yet fully established at synchrotron X-ray sources, despite accessibility for beamtime being far more readily available than at FEL X-ray sources. Furthermore, synchrotron X-ray sources are highly suitable for achieving time resolutions of micro- or milliseconds. The crystallographic phase problem also needs to be solved experimentally when applying serial crystallography. Through the work done in the course of this thesis, a method for gently introducing heavy atoms into micrometer sized crystals via diffusion was developed. This method negates the need for handling of the subsequent highly fragile, derivatized crystals by incorporating them directly into the viscous, lipidic cubic phase carrier material used to inject the crystals into the X-ray beam. Particularly membrane proteins, common drug targets, do not lend themselves to conventional macromolecular crystallography techniques, due to the fact that they often crystallize in showers of micro-crystals in a lipidic mesophase, as it mimics the native membrane environment. Due to the associated small crystal size used for serial crystallography experiments, the diffusion pathways through the crystals are decreased. Therefore this method also lends itself to mixing experiments, where a substrate or ligand is diffused into a protein crystal and is then turned over by the individual protein molecules without destroying the crystal structure. Towards the possibility of successfully applying this concept, temporal analysis of diffusion studies were conducted during the course of this thesis. Finally, a substrate could be successfully cross-linked into the substrate recognition site of the model protein in an attempt to visualize intermediate states during substrate turnover in the protein. This was then further applied serially, and a selected enzyme substrate-complex was analyzed applying serial crystallography. | en |
dc.description.abstract | Das ultimative Ziel der Strukturbiologie ist es, einzelne, biologische Partikel, wie zum Beispiel Viren, Ribosomen oder sogar Proteinmoleküle, mit atomarer Auflösung abzubilden, ohne die Proben mit aufwendigen Verfahren vorbereiten oder kristallisieren zu müssen. Die Entwicklung und Nutzung von Freien Elektronenlasern ist eine notwendige Bedingung, um dieses Ziel zu erreichen. In den letzten zehn Jahren wurde die Methodenentwicklung zur Einzelmolekülanalyse biologischer Objekte mittels kohärenter diffraktiver Röntgenbildgebung bereits zielorientiert vorangetrieben. Alle Verfahren basieren auf dem sogenannten ,,Diffraktion-vor-Zerstörung” Prinzip. Durch ultra-kurze, höchst brillante femtosekunden-R¨ontgenpulse können Diffraktionsdaten aufgenommen werden, bevor Strahlenschäden überhaupt entstehen. Die hohe Strahlensensitivität organischer Materie stellte bisher eine fundamentale Limitierung für die Analyse biologischer Objekte, wie zum Beispiel mithilfe von Röntgenstrukturanalyse oder Elektronenmikroskopie, dar. Die Messungen am FEL finden im Vakuum statt und setzen somit voraus, dass die zu analysierende Probe in Lösung eine homogene Verteilung aufweist und ohne Lösungsmittelrückstände in einer nahezu wasserfreien Umgebung gemessen werden kann. Im Rahmen dieser Arbeit wurden zwei Virusproben ausgewählt, die für eine weitergehende Methoden- und Algorithmenentwicklung geeignet sind. Es handelt sich um dsRNA-Mycoviren und dsDNA-Adenoviren, die charakterisiert und für zukünftige FEL Experimente vorbereitet wurden. Untersuchung durch Röntgenkleinwinkelbeugung war hier ein wesentlicher Bestandteil. Die neu etablierte Methodik der seriellen Kristallografie basiert ebenfalls auf dem Prinzip der ,,Diffraktion-vor-Zerstörung”. Mithilfe der seriellen Kristallografie können am FEL Röntgenlaser sogar Nanokristalle von biologischen Proben vermessen werden, die für das konventionelle Einkristallverfahren mit Synchrotronstrahlung nicht mehr zugänglich sind. Im Gegensatz dazu wird bei der seriellen Methode lediglich ein Diffraktionsbild pro Kristall aufgenommen, und die Diffraktionsbilder von tausenden, einzelnen Kristallen zu einem kompletten Datensatz zusammengefügt. Diese Methode ist inzwischen an FEL Röntgenlasern gut etabliert und darüber hinaus auch für Messungen mit konventioneller Synchrotronstrahlung adaptiert. Ein weiterer wesentlicher Vorteil der seriellen Kristallografie besteht darin, dass Messungen bei Raumtemperatur, also unter eher physiologischen Bedingungen, durchgeführt werden können. Messungen bei Raumtemperatur ermöglichen zukünftig weiterhin zeitaufgelöste Studien an Proteinen und Enzymen. Allerdings sind solche Experimente, vorallem an Synchrotronstrahlungsquellen, welche zugänglicher für Messzeiten sind als FEL Röntgenlaser, noch nicht hinreichend etabliert, obwohl sich insbesondere moderne Speicherringe für zeitaufgelöste Studien im mikro- und millisekunden Bereich gut eignen. Das sogenannte Phasenproblem der Kristallografie muss allerdings auch bei dem seriellen Ansatz experimentell gelöst werden. Im Rahmen dieser Arbeit wurde hierzu eine Methode entwickelt, bei der Schwermetall-Ionen besonders schonend in Protein mikrokristalle über Diffusion eingefügt werden können. Die Mikrokristalle liegen nach Anwendung dieser Methode bereits in einer viskosen, lipidisch-kubischen Phase als Trägermaterial vor und können so direkt für die Messungen genutzt werden. Insbesondere Membranproteine, wichtige Angriffspunkte bei der strukturbasierten Wirkstoffentwicklung, kristallisieren häufig ausschließlich in Form von Mikrokristallen in einer lipidisch kubischen Phase, da diese ihre natürliche hydrophobe Umgebung artifiziell nachbildet. Des Weiteren sind in Mikrokristallen die Diffusionszeiten für Substrate und Liganden stark reduziert. Dieses ist für zeitaufgelöste, diffusionsabhängige Enzym-Substrat Studien von Vorteil, weil die erforderliche Diffusionszeit deutlich kürzer sein muss, als die für den Substratumsatz benötigte Zeit. Um Diffusionszeiten in Abhängigkeit von der Kristallgröße zu bestimmen, wurden hier entsprechende Untersuchungen durchgeführt. Abschließend konnte erfolgreich ein Substrat in einem Proteinkristall quervernetzt werden, die Methodik wurde anschließend auf Mikrokristalle übertragen und seriell untersucht. Somit konnte ein ausgewählter Enzym-Substrat-Komplex mit Synchrotronstrahlung unter Anwendung eines seriellen Ansatzes analysiert werden. | de |
dc.language.iso | en | en |
dc.publisher | Staats- und Universitätsbibliothek Hamburg Carl von Ossietzky | |
dc.rights | http://purl.org/coar/access_right/c_abf2 | |
dc.subject | serielle Kristallographie | de |
dc.subject | zeit-aufgelöste Studien | de |
dc.subject | Phasierung | de |
dc.subject | Single Particle Imaging | en |
dc.subject.ddc | 540 Chemie | |
dc.title | Developing Methods towards the Structure Determination of Biological Particles using Crystallographic and Single Particle Imaging Techniques | en |
dc.title.alternative | Methodenweiterentwicklung für das Abbilden einzelner biologischer Partikel durch kohärente Diffraktion und Methodenentwicklung für serielle Kristallografie | de |
dc.type | doctoralThesis | |
dcterms.dateAccepted | 2018-04-13 | |
dc.rights.cc | No license | |
dc.rights.rs | http://rightsstatements.org/vocab/InC/1.0/ | |
dc.subject.bcl | 35.71 Biochemische Methoden | |
dc.type.casrai | Dissertation | - |
dc.type.dini | doctoralThesis | - |
dc.type.driver | doctoralThesis | - |
dc.type.status | info:eu-repo/semantics/publishedVersion | |
dc.type.thesis | doctoralThesis | |
tuhh.opus.id | 9124 | |
tuhh.opus.datecreation | 2018-05-04 | |
tuhh.type.opus | Dissertation | - |
thesis.grantor.department | Chemie | |
thesis.grantor.place | Hamburg | |
thesis.grantor.universityOrInstitution | Universität Hamburg | |
dcterms.DCMIType | Text | - |
tuhh.gvk.ppn | 1025065727 | |
dc.identifier.urn | urn:nbn:de:gbv:18-91244 | |
item.creatorOrcid | Botha, Sabine | - |
item.creatorGND | Botha, Sabine | - |
item.languageiso639-1 | other | - |
item.fulltext | With Fulltext | - |
item.advisorGND | Betzel, Christian (Prof. Dr.) | - |
item.grantfulltext | open | - |
Enthalten in den Sammlungen: | Elektronische Dissertationen und Habilitationen |
Dateien zu dieser Ressource:
Datei | Beschreibung | Prüfsumme | Größe | Format | |
---|---|---|---|---|---|
Dissertation.pdf | b47983024e593ac9ace3eacd57aab2fa | 22.39 MB | Adobe PDF | Öffnen/Anzeigen |
Diese Publikation steht in elektronischer Form im Internet bereit und kann gelesen werden. Über den freien Zugang hinaus wurden durch die Urheberin / den Urheber keine weiteren Rechte eingeräumt. Nutzungshandlungen (wie zum Beispiel der Download, das Bearbeiten, das Weiterverbreiten) sind daher nur im Rahmen der gesetzlichen Erlaubnisse des Urheberrechtsgesetzes (UrhG) erlaubt. Dies gilt für die Publikation sowie für ihre einzelnen Bestandteile, soweit nichts Anderes ausgewiesen ist.
Info
Seitenansichten
709
Letzte Woche
Letzten Monat
geprüft am 03.04.2025
Download(s)
400
Letzte Woche
Letzten Monat
geprüft am 03.04.2025
Werkzeuge