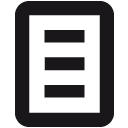
Titel: | Scaling and balancing carbon fluxes in a heterogeneous tundra ecosystem of the Lena River Delta | Sonstige Titel: | Skalierung und Bilanzierung von Kohlenstoffflüssen in einem heterogenen Tundra-Ökosystem des Lena Deltas | Sprache: | Englisch | Autor*in: | Rößger, Norman | Schlagwörter: | Eddy Kovarianz; Repräsentativität; stufenweise Regression; neuronales Netzwerk; Footprint; Sensor location bias | GND-Schlagwörter: | Arktis Tundra Dauerfrostboden Mikrometeorologie Heterogenität Treibhausgas Methan Kohlendioxid Photosynthese Atmung Gasaustausch |
Erscheinungsdatum: | 2018 | Tag der mündlichen Prüfung: | 2018-04-27 | Zusammenfassung: | The Arctic tundra has been a carbon sink through the comprehensive accumulation of carbon in permafrost-affected soils over thousands of years. This carbon may be remobilised in the course of climate change, which occurs more pronounced in the Arctic region than on the global average. With growing concern about rising emissions of the greenhouse gases methane and carbon dioxide, the current and prospective carbon turnover of the terrestrial Arctic is regularly assessed. However, these assessments are associated with large uncertainties, which can (inter alia) be ascribed to both the general shortage of flux data from the vast and sparsely inhabited Arctic region, and the typically high spatiotemporal variability of carbon fluxes in tundra ecosystems. Thus, this study aims at providing robust carbon budgets from a Siberian tundra landscape that has not yet been investigated: an active flood plain situated in the Lena River Delta. Applying the eddy covariance methodology, methane and carbon dioxide fluxes were determined during the growing seasons 2014 and 2015. These fluxes exhibited a great deal of temporal variability, which was, besides seasonal variation, largely the result of the pronounced spatial variability of soil and vegetation characteristics within the footprint. In order to explain this flux variability, the vegetation of the entire flood plain was classified utilising a high-resolution orthomosaic. In this process, three vegetation classes were designated accounting for shrubs (large Salix spp.), sedges (Carex spp., Eriophorum sp., Equisetum sp.) and intermediate (low Salix spp., Carex spp.) vegetation. These classes were well coupled with environmental variables such as soil moisture, moss properties and active layer depth. Hence, the vegetation served as an integrated proxy for potential soil-related flux drivers, whose contribution to the flux signal permanently varied according to the shifting source area. Applying footprint information in the form of relative contributions of vegetation classes to the flux signal facilitated the diagnostic modelling of the observed fluxes in the heterogeneous footprint to a large extent. The modelling of the methane fluxes was based on three data-driven approaches: the automatically operating algorithms stepwise regression as well as artificial neural network, and a mechanistic model, which utilises exponential relationships between the methane flux and both flux drivers soil temperature and friction velocity. On balance, the neural network exhibited the best model performance. However, verifying the models’ generalisability revealed that the mechanistic model provided the most predictive power suggesting that this model best captured the causality between the methane flux and its drivers. After integrating the accordingly gap-filled time series, all models yielded footprint budgets that were very similar in magnitude; e.g. the footprint budget estimated with the mechanistic model amounted to 96.1 mmol m^-2 (2014) and 104.3 mmol m^-2 (2015). The modelling of the carbon dioxide fluxes was based on another mechanistic model, which simultaneously computed the respiratory loss and the photosynthetic gain. This model was run with both flux drivers air temperature and irradiance, and it required a comprehensive calibration process, in the course of which its parameterisation repeatedly varied. Aggregating the time series after accordingly filling the data gaps yielded footprint budgets of 3.81 mol m^-2 (2014) and 5.27 mol m^-2 (2015). For assessing the carbon sink/source strength of the tundra landscape in question, the footprint budgets of carbon dioxide were mostly applicable on account of the frequently minor differences between the flux rates of the vegetation classes. The footprint budgets of methane, however, lacked representativeness due to the sensor location bias, i.e. their strong dependence on tower location, measurement height and wind field conditions. For an unbiased appraisal of the sink/source function, a robust greenhouse gas budget of the entire flood plain was hence estimated. Since both mechanistic models for methane carbon dioxide were additive models that account for the flux dynamics of each vegetation class, the footprint fluxes could be decomposed. For methane, this downscaling procedure partitioned the observed fluxes with a seasonal mean of 0.012 μmol m^-2 s^-1 into three individual vegetation class fluxes with the following mean rates: 0.0004 μmol m^-2 s^-1 for shrubs, 0.052 μmol m^-2 s^-1 for sedges and 0.018 μmol m^-2 s^-1 for intermediate vegetation. While these individual methane fluxes respectively featured similar seasonal courses for both years, the downscaling procedure unveiled a differing seasonality of the individual fluxes for carbon dioxide. In this process, the observed fluxes with a seasonal mean of 0.61 μmol m^-2 s^-1 were separated into two individual fluxes, whose mean rates were as follows: 0.79 μmol m^-2 s^-1 for shrubs and 0.53 μmol m^-2 s^-1 for both sedges and intermediate vegetation together. During the comparatively warm spring in 2014, the shrubs exhibited a considerably greater net uptake than the sedges. On the contrary, shrubs and sedges displayed a more similar net uptake during the climatologically usual measurement period in 2015. This varying ratio of net uptakes between the vegetation classes indicates a seasonally varying implication of the sensor location bias; i.e. climatologically unusual conditions might adversely affect the representativeness of a footprint due to sharpened contrasts between vegetation classes. The downscaled fluxes in turn formed the initial data for the upscaling procedure, which was based on the area-weighted projection of the cumulative vegetation class-specific fluxes. While the methane release for the entire flood plain was estimated at 0.171 Mmol and 0.177 Mmol for 2014 and 2015, respectively, the carbon dioxide net uptake was estimated at 4.42 Mmol and 6.17 Mmol. The conversion of the methane budgets according to their global warming potential yielded a greenhouse gas sink strength of 2.31 ± 0.64 Mmol CO2 eq in 2014 and 3.98 ± 0.78 Mmol CO2 eq in 2015. This carbon turnover, which is larger than at many other Arctic sites, can be attributed to the annual spring flood and the associated fluvial deposition of both fresh organic matter and nutrients, which enhance productivity and microbial breakdown. Alternatively, the footprint budgets were simply projected on the flood plain: these subsidiary budgets of methane and carbon dioxide were, respectively, on average roughly 42 % and 4 % lower than the adequately estimated flood plain budgets utilising the preceding flux decomposition. Thus, the simple, commonly applied upscaling method overestimated the greenhouse gas sink strength in great measure. This comparison emphasises the necessity of considering the surface heterogeneity for modelling and balancing carbon flux dynamics in heterogeneous tundra regions and potentially other biomes with heterogeneous ecosystems, which the advanced scaling methodology of this study could also be applicable to. Die arktische Tundra ist seit Jahrtausenden eine Kohlenstoffsenke auf Grund der Einlagerung beträchtlicher Mengen von Kohlenstoff in den Permafrostböden. Dieser gespeicherte Kohlenstoff ist von großer Bedeutung für das globale Klima, da dessen Freisetzung in Form von Methan und Kohlenstoffdioxid den in der Arktis ohnehin verstärkten Klimawandel weiter beschleunigen könnte. Grundlage zur Schätzung dieser Freisetzung in Gegenwart und Zukunft bilden Kohlenstoffbilanzen, welche jedoch meist mit großen Ungenauigkeiten behaftet sind. Diese Ungenauigkeiten können insbesondere auf den generellen Mangel von notwendigen Eingangsdaten aus den weiten und dünn besiedelten Gegenden der Arktis zurückgeführt werden, aber auch die typischerweise hohe räumlich-zeitliche Variabilität von Kohlenstoffflüssen in der Tundra spielt in diesem Zusammenhang eine übergeordnete Rolle. Ziel dieser Arbeit ist daher, belastbare Kohlenstoffbilanzen eines bisher unerforschten Ökosystems in der sibirischen Tundra zu schätzen: eine Überflutungsebene im Lena Delta. Unter Anwendung der Eddy Kovarianz Methode wurden während der Vegetationsperiode in 2014 und 2015 Methan- und Kohlenstoffdioxidflüsse bestimmt. Diese Flüsse wiesen eine hohe zeitliche Variabilität auf, welche sich, neben den jahreszeitlichen Schwankungen, insbesondere aus der räumlichen Variabilität der Boden- und Vegetationseigenschaften innerhalb des Footprints (d.h. des beprobten Bereichs um den Messturm) ergab. Zur Erklärung dieser Variabilität wurde die Vegetation der gesamten Überflutungsebene mit Hilfe eines hochaufgelösten Orthomosaiks klassifiziert. Die dabei verwendeten Klassen bilden Büsche (hohe Salix spp.), Seggen (Carex spp., Eriophorum sp., Equisetum sp.) und Übergangsvegetation (niedrige Salix spp., Carex spp) ab. Diese drei Klassen waren eng mit den Parametern Bodenfeuchte, Mooseigenschaften und Auftautiefe verbunden, sodass die Vegetation als eine integrierend stellvertretende Größe für diese Steuerparameter diente. Deren sich ständig verändernde Zusammensetzung im Flusssignal konnte mittels Footprint-Informationen in Form der relativen Anteile der Vegetationsklassen im Flusssignal abgebildet werden, was die diagnostische Modellierung der Flüsse in dem heterogenen Footprint in hohem Maße gefördert hat. Die Modellierung der Methanflüsse basierte auf drei Daten-getriebenen Ansätzen: die zwei automatisierten Algorithmen stufenweise Regression und künstliches neuronales Netzwerk sowie ein mechanistisches Modell, das exponentielle Zusammenhänge zwischen Methanfluss und den beiden Steuergrößen Bodentemperatur und Schubspannungsgeschwindigkeit verwendet. Dabei wies das neuronale Netzwerk die höchste Modellgüte auf. Die Überprüfung der Generalisierbarkeit der Modelle zeigte jedoch, dass das mechanistische Modell die meiste Vorhersagekraft besitzt, sodass dieses Modell den kausalen Zusammenhang zwischen Methanfluss und Steuergrößen am besten erfasst hat. Die nach Aufsummierung der entsprechend gefüllten Zeitreihen erhaltenen Bilanzen waren für alle drei Modelle sehr ähnlich; so betrugen zum Beispiel die mit dem mechanistischen Modell geschätzten Footprint-Bilanzen 96.1 mmol m^-2 (2014) und 104.3 mmol m^-2 (2015). Die Modellierung der Kohlenstoffdioxidflüsse basierte auf einem weiteren mechanistischen Modell, welches die respiratorische Freisetzung und die photosynthetische Bindung von Kohlenstoffdioxid gleichzeitig berechnete. Dieses Modell wurde mit den beiden Steuergrößen Lufttemperatur und Strahlungsintensität angetrieben. Weiterhin erforderte es eine umfangreiche Kalibrierung, in deren Verlauf acht Modellparameter ausgegeben wurden, welche ökophysiologische Eigenschaften charakterisierten. Die Aufsummierung der entsprechend lückengefüllten Zeitreihen ergab Footprint-Bilanzen von 3.81 mol m^-2 (2014) und 5.27 mol m^-2 (2015). Zur Beurteilung der Senken- bzw. Quellenfunktion dieser heterogenen Tundra waren die Footprint-Bilanzen für Kohlenstoffdioxid meistens geeignet, da die Unterschiede zwischen den Flüssen der Vegetationsklassen vorwiegend geringerer Größenordnung waren. Den Footprint-Bilanzen für Methan fehlte es jedoch an Repräsentativität auf Grund ihrer starken Abhängigkeit von der Position des Flussturms, der Messhöhe und den Windfeldbedingungen. Für eine unverfälschte Bewertung der Senken- bzw. Quellenstärke wurde daher eine belastbare Gesamtkohlenstoffbilanz für die gesamte Überflutungsfläche geschätzt. Da die mechanistischen Modelle für Methan und Kohlenstoffdioxid additive Modelle sind, welche die Dynamik der Flüsse in den einzelnen Vegetationsklassen erfassen, konnten die Footprint-Flüsse zerlegt werden. Diese Runterskalierung der beobachteten Methanflüsse, welche im saisonalen Mittel 0.012 μmol m^-2 s^-1 betrugen, ergab folgende mittleren Flussraten für die einzelnen Vegetationsklassen: 0.0004 μmol m^-2 s^-1 für Büsche, 0.052 μmol m^-2 s^-1 für Seggen und 0.018 μmol m^-2 s^-1 für die Übergangsvegetation. Während diese einzelnen Methanflüsse in beiden Jahren jeweils einem ähnlichen Verlauf folgten, ergab die Flusszerlegung eine partiell unterschiedliche Saisonalität der Kohlenstoffdioxidflüsse für die einzelnen Vegetationsklassen. Die beobachteten Flüsse, welche im saisonalen Mittel 0.61 μmol m^-2 s^-1 betrugen, wurden in zwei einzelne Flüsse mit folgenden mittleren Flussraten aufgetrennt: -0.79 μmol m^-2 s^-1 für Büsche und 0.53 μmol m^-2 s^-1 für Seggen und Übergangsvegetation zusammen. Während des vergleichsweise sehr warmen Frühlings in 2014 wiesen die Büsche eine wesentlich höhere Nettoaufnahme als die Seggen auf. Im Gegensatz dazu zeigten Büsche und Seggen eine ähnliche Nettoaufnahme während des klimatologisch durchschnittlichen Messzeitraums in 2015. Diese variable Ähnlichkeit zwischen den Nettoaufnahmen beider Vegetationsklassen weist auf eine Wetter-induzierte, zeitliche Einschränkung der Repräsentativität des Footprint hin. Die runterskalierten Flüsse bildeten den Ausgangspunkt für die Hochskalierung, welche auf einer Flächen-gewichteten Projektion der aufsummierten Flüsse aller Vegetationsklassen basierte. Während die Methanabgaben der gesamten Überflutungsebene auf jeweils 0.171 Mmol und 0.177 Mmol für 2014 und 2015 geschätzt wurden, betrugen die geschätzten Kohlenstoffdioxidaufnahmen jeweils 4.42 Mmol und 6.17 Mmol. Die Umrechnung der Methanbilanzen zur Berücksichtigung deren Treibhauspotentials ergaben eine robuste Schätzung der Treibhausgasbilanz von 2.31 ± 0.64 Mmol CO2 eq in 2014 und 3.98 ± 0.78 Mmol CO2 eq in 2015. Dieser im Vergleich zu vielen anderen arktischen Standorten hohe Kohlenstoffumsatz kann auf das jährliche Frühjahrshochwasser zurückgeführt werden, in dessen Verlauf frisches organisches Material und Nährstoffe abgelagert werden, welche die Aufnahme und Freisetzung von Treibhausgasen fördern. Alternativ wurden Footprint-Bilanzen einfach auf die Überflutungsebene projiziert: die auf diese Weise geschätzten Methan- und Kohlenstoffdioxidbilanzen waren jeweils um 42 % und 4 % niedriger als die belastbaren Kohlenstoffbilanzen, welche mit der vorangegangenen Flusszerlegung geschätzt wurden. Demzufolge hat diese einfache sowie übliche Hochskalierung der Footprint-Bilanzen die Senkenfunktion für Treibhausgase in hohem Maße überschätzt. Dieser Vergleich verdeutlicht die Notwendigkeit der Berücksichtigung der heterogenen Unterlage bei der Modellierung und Bilanzierung von Kohlenstoffflüssen in heterogenen Tundren und darüber hinaus anderen Ökosystemen mit heterogener Unterlage, für welche die in dieser Studie entwickelte Skalierungsmethodik ebenfalls Anwendung finden kann. |
URL: | https://ediss.sub.uni-hamburg.de/handle/ediss/7966 | URN: | urn:nbn:de:gbv:18-94792 | Dokumenttyp: | Dissertation | Betreuer*in: | Kutzbach, Lars (Prof. Dr.) |
Enthalten in den Sammlungen: | Elektronische Dissertationen und Habilitationen |
Dateien zu dieser Ressource:
Datei | Beschreibung | Prüfsumme | Größe | Format | |
---|---|---|---|---|---|
Dissertation.pdf | 95b86cb73995cf2fb4449964897357b7 | 14.73 MB | Adobe PDF | Öffnen/Anzeigen |
Diese Publikation steht in elektronischer Form im Internet bereit und kann gelesen werden. Über den freien Zugang hinaus wurden durch die Urheberin / den Urheber keine weiteren Rechte eingeräumt. Nutzungshandlungen (wie zum Beispiel der Download, das Bearbeiten, das Weiterverbreiten) sind daher nur im Rahmen der gesetzlichen Erlaubnisse des Urheberrechtsgesetzes (UrhG) erlaubt. Dies gilt für die Publikation sowie für ihre einzelnen Bestandteile, soweit nichts Anderes ausgewiesen ist.
Info
Seitenansichten
426
Letzte Woche
Letzten Monat
geprüft am 03.06.2025
Download(s)
185
Letzte Woche
Letzten Monat
geprüft am 03.06.2025
Werkzeuge